Underground Utilities - Time-Domain Electromagnetic (TDEM) Method
Target of Investigation
The time-domain electromagnetic (TDEM) method is a geophysical nondestructive evaluation (NDE) technique that uses electromagnetic (EM) signals to detect and identify subsurface anomalies based on changes in electric conductivity or magnetic susceptibility within an area of investigation. Although the TDEM method is generally used in surveys to determine the electrical properties of a given area’s geology, the ability to induce electrical currents in metallic subsurface targets allows this technology to be used to detect buried utilities. The systems for the TDEM method tend to be robust in the face of confounding surface conditions relative to the frequency-domain electromagnetic (FDEM) method. With expert handling and data interpretation, these systems can provide utility detection results at reasonable acquisition speeds and with portable hardware. Different pipe types have varying responses to the use of the TDEM method, which must be considered in its deployment, as presented in table 1.
Table 1. Summary of TDEM effectiveness for different materials.
Material | Detects | Response Strength |
Metal/steel | Yes | Strong |
Plastic/polyvinyl chloride (PVC) | No1 | None |
Electrical line | Yes | Medium-low |
Fiber optic | No1 | None |
Concrete | No1 | None |
Description
TDEM uses a coil of wire connected to a transmitter. The transmitter is powered by an electrical current that generates a primary magnetic field at its center. The current is then shut off, collapsing the magnetic field, which then induces a propagating ring of eddy currents into the subsurface via EM induction. These eddy currents generate a decaying secondary magnetic field that propagates back to the surface, where it is detected and measured. A secondary coil (or in some models, the same coil as the transmitter) is connected to a receiver that measures the voltage induced over time by the decaying secondary magnetic fields. The decaying voltage is recorded in discrete intervals called time gates. TDEM systems designed for metal detection record the voltage at late time gates because subsurface metal will generate a secondary magnetic field for a much longer time than that of geological materials. The data acquisition system typically presents the user with a visual display of the various time gates as well as an audio signal. These systems may be installed in a wheeled cart and use a physical encoder. A Global Positioning System (GPS) device is typically used to stream positional data into the acquisition system and connects TDEM measurements with their respective positions in a survey area.
The transmitter, receiver, and data acquisition tend to be operator‑portable, with the coils placed approximately 1 ft above the ground surface. Local anomalies observed in the measurements can be used to identify the presence of a buried ferrous or nonferrous utility. If a buried utility and its material type can be distinguished from the surrounding geology by one of these anomalies, TDEM is an accepted technology for locating and mapping subsurface utility lines. As with most NDE methods, TDEM is best used as a complementary tool in the engineer’s toolbox rather than as a stand-alone technology that provides a complete solution to every problem.
Traditional deployments of TDEM use a single set of transmitter/receiver coils within a portable cart for scans to be gathered on foot. Recent advancement includes development of a multichannel, vehicle-based system for use on roadways (figure 1).(1,2,3) The vehicle-based TDEM method collects data at higher speeds and efficiency than traditional approaches because data is gathered from a moving vehicle with multiple sensor arrays working in parallel to minimize the number of collection passes. The major drawback, however, is the initial cost of the hardware unit and data acquisition software relative to simpler traditional systems.
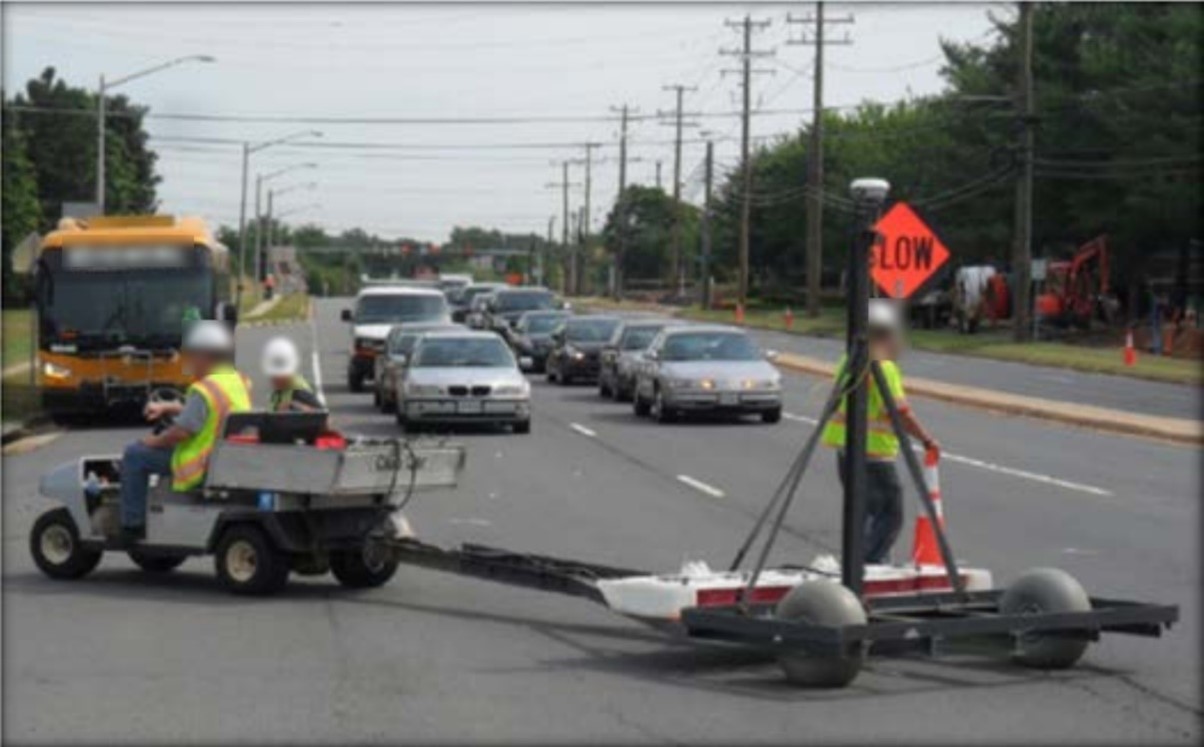
Figure 1. Photo. An early prototype of a TDEM system being tested during a previous Strategic Highway Research Program 2 study.(1)
Physical Principle
TDEM is based on the principles of eddy currents. A transmitter coil connected to a direct current source emits a primary magnetic field. As the field is turned off, eddy currents produce an induced, secondary magnetic field, directly below the coil (figure 2). The magnetic field flows to the surface with slightly different properties when it encounters a buried object with different EM properties from the surrounding soil. A receiver at the ground surface measures the response at several time gates. By normalizing (or zeroing) all the time gates at a location with no subsurface metal, only variations due to the presence of metallic objects are recorded. Elevated signal responses indicate subsurface metal. A large difference in signal strength between early and late time gates typically indicates small and shallow metallic objects while a smaller difference indicates deeper, larger metallic objects. TDEM uses a periodic transmitter signal—but it focuses on the decay of the induced signal in the buried target. This TDEM method reduces its ability to be tuned to adapt to different conditions but makes the system more robust overall in general conditions.(5)
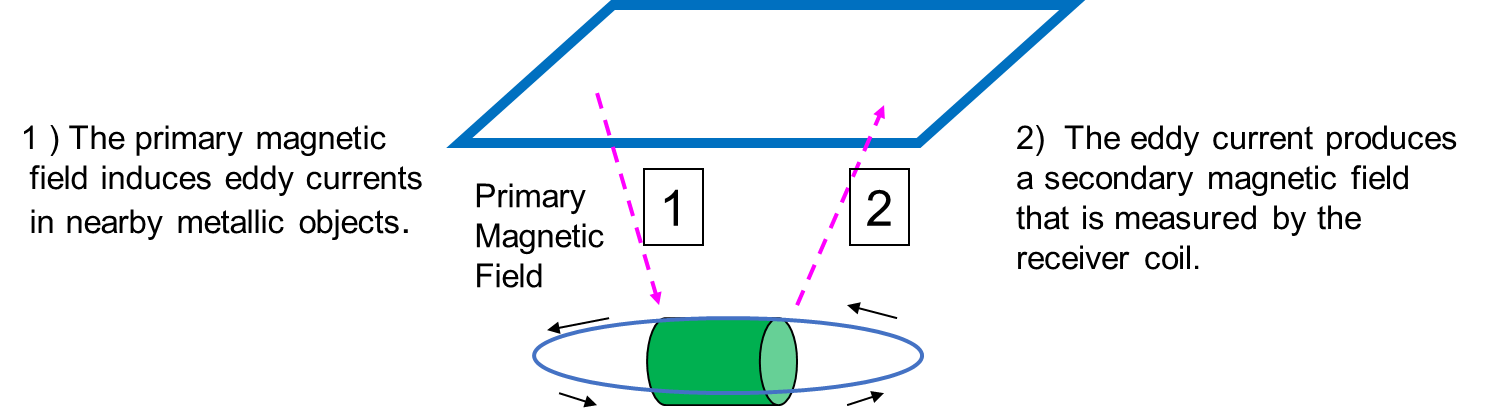
Figure 2. Illustration. Principles of eddy currents as applied to TDEM.
EM waves cannot penetrate metals (e.g., steel pipes and metal sheets), which means that TDEM cannot image any features below a metal object or layer. Local metallic surface objects, such as fences, vehicles, reinforced concrete, or sewer tops, tend to saturate the late time gates. This effect impacts the ability to interpret the EM wave field, resulting in significant limitation in utility location in such environments.(4) In addition, TDEM cannot detect fiber optic and polyvinyl chloride (PVC) pipes on their own, and recent investigations of TDEM for utility location were also unsuccessful in locating nonferrous electrical lines.(1) Some utility providers will overcome this limitation by attaching a metallic tracer cable alongside the nonmetallic utility line to provide an accessible detection method for the TDEM instrument. However, in otherwise dry and nonclay conditions, TDEM can be an effective tool for locating buried utilities made of materials of sufficiently distinct electric and/or magnetic properties from the surrounding material. TDEM systems are particularly useful at sites with many unknown or abandoned utilities with no surface appurtenances or where access to manholes may be restricted or unpractical.
Data Acquisition
Data collection with TDEM should be carried out by an experienced and qualified operator. The following list provides the general steps needed to achieve quality results during a TDEM utility investigation.
Fieldwork Preparation
- Identify an area of interest for the investigation with an appropriate coordinate system laid out using semipermanent marking paint to identify its extents and major features. This coordinate system should have the following features:
- A well-defined, easily identifiable, and reasonably permanent origin from which all lateral measurements across the test area can be referenced.
- An intuitive selection of the x- and y-axis orientation for the coordinate system.
- Indications at regular intervals along each grid axis to denote the distance from the reference origin to identify the start location and orientation of a given scan.
- Document the coordinate system with a sketch from the field showing the appropriate measurements of the coordinate system’s extents, reference origin, x- and y-axis orientation, and a north arrow. In addition, document the weather conditions, observed soil conditions, and other relevant details for context when analyzing the gathered data.
- Complete the definition of the test area extents for quality control of the data positioning when using TDEM in combination with GPS, where the extents are locked in using appropriate GPS equipment and relevant procedures for coordinate lock-in operations.
- Determine and document the intervals along the test area where the scans will be gathered, forming a test grid. Scans should be taken in both the x- and y-direction along the grid because TDEM systems are generally polarized so they can only detect pipes oriented perpendicular to the direction of scanning. A typical spacing may be 5 ft (1.5 m) in either direction, with a 2-ft (0.6 m) spacing for high-resolution imaging.
- Develop and follow a notes package that records the start position, orientation, and relevant TDEM filename for each scan gathered to use in analyzing and reporting the data.
System Operation
- Connect all appropriate system elements, such as the TDEM instrument, data acquisition system, and GPS system.
- Power on the TDEM system based on the manufacturer’s instructions and review all system components to ensure it can send and receive data with the data acquisition system.
- Ensure the GPS system has enough satellite location locks to take precise measurements of its position.
Field Data Collection
- Collect data according to the manufacturer’s instructions on system operations, beginning with the instrument aligned on the appropriate start location on the coordinate system. Scan collection should be completed systematically along the test grid in both directions, with notes and documentation gathered where appropriate.
- Ensure data collection settings balance the transmitter frequency, sampling rate, rate of travel, penetration depth, and imaging precision, where lower transmitter frequencies and sampling rates can translate to higher rates of travel and penetration depths at the cost of precision.
- Review data in realtime to ensure data are actually being gathered and meet a minimum standard of quality.
- Mark features onsite, if desired, with marking paint as they are discovered during scanning.
- Perform a quality-control review of onscreen output for permanent storage (hard drive, flash drive, memory stick, or another medium) after completing the scanning.
Data Processing
Some modern software packages may have tools for presenting and mapping anomalies in measurements as a function of their GPS position. However, the traditional output of TDEM data acquisition is often a table in which each row represents a date- and time-stamped measurement with columns for relevant equipment settings, conductivity measurements, and GPS positioning.
Data Interpretation
By using typical computer applications for plotting, individual line scans can be presented as either scatterplots of measurements or two-dimensional contour maps where the position in the map is set by the GPS position, with the contour and color scaling representing the respective measurement. Analyzing the results from several time gates for complete data interpretation is recommended. In either presentation approach, the analyst’s goal when evaluating the data is to identify local anomalies where a significant change in response is observed. These anomalies can then be mapped across the area of investigation to identify consistent patterns where they align to form what appears to be a continuous subsurface structure, such as a buried utility.
Advantages
- Well-established field data collection processes.
- Commercially available hardware that can be configured from 1- to 4-m (3.3- to 13-ft) width.
- Rapid-test method with an operator-portable hardware system.
- Reliable and repeatable techniques, especially for utilities made of ferrous and nonferrous metals.
- Real-time marking of utility features when used by an expert operator.
Limitations
- Advanced expertise and training needed for data collection, processing, and interpretation.
- Data are negatively influenced by the presence of surface metallic objects such as fences, vehicles, dumpsters, or sewer lids.
- PVC, fiber optics, clay, or other nonconductive and nonmagnetic utilities cannot be detected.
- Calibration with other NDE methods and ground-truth activities required, especially when depth-of-feature measurements are desired.
References
- Young, G. N. and C. M. Kennedy. 2015. Utility-Locating Technology Development Using Multisensor Platforms. Report No. S2-R01B-RW-1. Washington, DC: The National Academies Press. https://www.trb.org/Publications/Blurbs/171470.aspx, last accessed February 15, 2023.
- American Association of State Highway and Transportation Officials. n.d. “Improving Coordination with Utilities (R01A, R01B, R15B)” (web page). https://shrp2.transportation.org/Pages/UtilityRelatedProducts.aspx, last accessed February 15, 2023.
- Federal Highway Administration. n.d. “Advancing Technologies to Help Agencies Detect Subsurface Utilities. Utility Investigation Technologies (R01B)”(web page). https://www.fhwa.dot.gov/goshrp2/Solutions/Renewal/R01B/Utility_Investigation_Technologies, last accessed February 15, 2023.
- American Society of Civil Engineers. 2002. Standard Guidelines for the Collection and Depiction of Existing Subsurface Utility Data. New York, NY: American Society of Civil Engineers.
- Wightman, W. E., F. Jalinoos, P. Sirles, and K. Hanna. 2004. Application of Geophysical Methods to Highway Related Problems. Report No. FHWA-IF-04-021. Washington, DC: Federal Highway Administration. https://intrans.iastate.edu/app/uploads/sites/10/2019/01/dot_49856_DS1.pdf, last accessed February 15, 2023.