Tunnel - Guided Wave Ultrasonics (GWU)
Target of Investigation
Ultrasonic guided waves can be used to detect cracks and corrosion damage in steel. Guidedwave ultrasonic technology can be applied to plates and plate-like structures, such as steel beams, girders, tubular pipes, and truss members, as well as to steel tunnel linings, metal tiles, and other plate-like tunnel components.
Description
Guided-wave ultrasonics is a technology that can be used to detect damage. Lamb waves, guided by the dimensions of the testing structure, can be used to detect cracks and corrosion damage in plates or plate-like structures. These guided waves can be broadcast for distances up to 100 m (328 ft) from a single transducer.(1) This distance enables the technology to reach inaccessible areas of a plate. For example, guided waves can propagate through areas of a plate that are coated, embedded in concrete, covered by insulation, or located behind structural features that block access to the surface. This technology has been applied on an experimental basis to detect fatigue cracks in steel bridge members and orthotropic decks.(2–5) The technology has the potential to detect areas of section loss or cracking in steel tunnel linings, metal tiles, or other plate-like components with limited accessibility. Researchers face significant challenges when geometries are complex or components are present that can obscure signals from damaged areas.
Physical Principle
The term guided-wave ultrasonics commonly refers to the use of Lamb waves that propagate within the boundaries of a plate. Lamb waves propagate in an infinite number of possible modes with either symmetric or antisymmetric displacements, as shown in figure 1. Zero-order modes carry most of the wave energy and, therefore, are the focus of many approaches for detecting damage.(6) Symmetric modes are commonly referred to as extensional modes because the average movement through the plate thickness is aligned with the wave propagation direction. Antisymmetric modes are commonly referred to as flexural modes because the average displacements through the plate thickness are orthogonal to the direction of propagation.
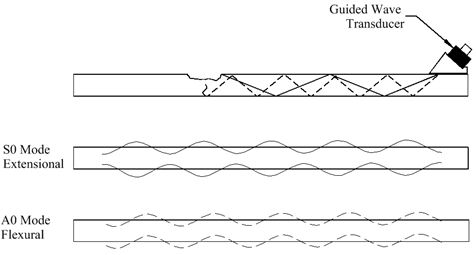
© 2016 Glenn Washer.
The wavelengths and modes that propagate are functions of the plate thickness. Zero-order modes have a nascent frequency of zero, meaning that these waves can propagate at any wavelength in a plate. Higher-order modes exhibit a cutoff frequency below which the waves cannot propagate in a plate. Different modes exhibit different displacement characteristics. All modes are dispersive, meaning the velocity of the wave varies as a function of the wavelength (frequency). Because Lamb waves are bounded and guided by plate boundaries, they can propagate over long distances. In contrast to traditional ultrasonic testing, which uses highfrequency pulses and wavelengths that are much smaller than the dimensions of the part being tested, guided waves typically carry energy associated with long wavelengths that resonate in the plate and exhibit low-attenuation characteristics. The frequencies of ultrasonic guided waves that are of interest for civil infrastructure vary, depending on the thickness of the element of interest, from 10 kHz to several hundred kHz. As a result, Lamb waves are complex and can be tuned to a particular application depending on the thickness of the plate, the type of damage of interest, and the distance from the source transducer to the area under inspection.
Data Acquisition
Guided-wave ultrasonic systems typically consist of ultrasonic transducers energized by a transmitter to launch acoustic waves of a certain central frequency. Waves may be launched into the plate at critical angles intended to excite a particular mode or modes within the plate. The choice of mode and frequency will control the strength of the reflection of Lamb waves from a sought defect. Transducer arrays, consisting of several transducers positioned geometrically to excite certain modes, may also be used. Electromagnetic acoustic transducers are sometimes used because they can excite certain modes based on the geometric configuration of the transducer.
Transducers may be arranged in a pitch–catch or pulse–echo configuration. In a pitch–catch configuration, one transducer acts as a transmitter to launch the acoustic wave into the material, and the second transducer acts as the receiver, detecting the acoustic wave after it has propagated through some portion of the material being tested. In a pulse–echo configuration, a single transducer acts as both transmitter and receiver, detecting waves reflected from discontinuities in the material being tested (figure 1). In both configurations, received waveforms are digitized and stored for analysis.
Data Processing
Data processing typically consists of amplifying received waveforms for analysis and interpretation in the time domain. To isolate specific wave modes, the received signals are often filtered to remove energy in unwanted frequency ranges. Fast Fourier transforms can be used to analyze the received signals in the frequency domain.
Data Interpretation
Signals can be analyzed in the time domain and/or the frequency domain. In the time-domain analysis, the arrival times and amplitude variations of the acoustic waves are used to detect features, such as section loss and cracks, in the material being tested. This is similar to the objectives of conventional pulse–echo ultrasonic methods.(3) In the frequency-domain analysis, the amplitude spectrums (the variation in amplitude with frequency) of captured time-domain signals are analyzed to identify anomalies in the member, such as thinning or cracking.(7) Figure 2 provides an example of the peak frequency shift in a guided wave propagated through a portion of material with a 10-percent section loss.
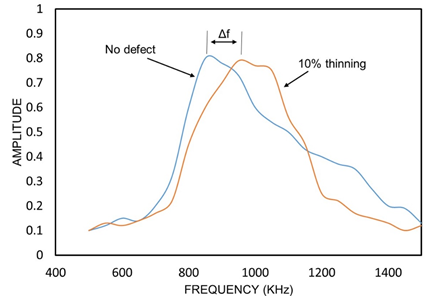
Δf = the difference in peak frequency.
Figure 2. Graph. The peak frequency shift of a guided wave as a result of plate thinning.
Advantages
Advantages of guided-wave ultrasonics include the following:
- Large areas can be tested from a single location.
- Frequency and mode tuning can be used to establish wave resonances that improve detection capabilities for certain defect types and their orientations.
- Plates with coatings, such as insulation, can be inspected.(8)
- The testing is cost effective.
Limitations
Limitations of guided-wave ultrasonics include the following:
- Features, such as stiffeners and attachments, result in complex responses and may inhibit long-range inspection.
- This technology is better suited for screening than for detailed characterization of defects.
- Signal analysis and test setup are complex.
- Few standards or guides for implementation exist.
References
- Matzkanin, G.A. and Yoken, H.T. (2007). “Nondestructive Evaluation Techniques for Detecting Hidden Corrosion.” AMMTIAC Quarterly, 2(2), pp. 3–6, Advanced Materials, Manufacturing, and Testing Information Analysis Center, Alexandria, VA.
- Macías, G.G.R. (1997). Long range crack detection in the welded girder-diaphragm connection, Ph.D. Thesis, New Mexico State University, Las Cruces, NM.
- Woodward, C. and McGarvie, A. (2000). “Long Range Ultrasonic Fatigue Detection.” Structural Materials Technology Ⅳ Conference Proceedings, pp. 267–271, Technomic Publishing Co., Inc., Atlantic City, NJ.
- Oppenheim, I., Greve, D., and Tyson, N. (2006). “Lamb Wave Behavior in Bridge Girder Geometries.” Smart Materials and Structures, 6174, pp. 759–766, International Society for Optics and Photonics, Bristol, United Kingdom.
- Pahlavan, L. and G. Blacquière. (2016). “Fatigue crack sizing in steel bridge decks using ultrasonic guided waves.” NDT & E International, 77, pp. 49–62, Elsevier, Amsterdam, Netherlands.
- Wilcox, P., Lowe, M., and Cawley, P. (2001). “Mode and Transducer Selection for Long Range Lamb Wave Inspection.” Journal of Intelligent Material Systems and Structures, 12(8), pp. 553–565, Sage Journals, Thousand Oaks, CA.
- Tuzzeo, D. and Di Scalea, F.L. (2001). “Noncontact Air-Coupled Guided Wave Ultrasonics for Detection of Thinning Defects in Aluminum Plates.” Journal of Research in Nondestructive Evaluation, 13(2), pp. 61–77, Taylor & Francis, London, United Kingdom.
- Rose, J.L. (2002). “A Baseline and Vision of Ultrasonic Guided Wave Inspection Potential.” Journal of Pressure Vessel Technology, 124(3), pp. 273–282, American Society of Mechanical Engineers, New York, NY.