Tunnel - Galvanostatic Pulse Measurement (GPM)
Target of Investigation
Knowing the corrosion rate of a concrete member is important for determining how fast the member is deteriorating. The galvanostatic pulse method (GPM) can estimate the corrosion rate of rebars in reinforced concrete members. This rate cannot be determined by electrical resistivity (ER) or half-cell potential (HCP) testing. GPM is better than HCP measurement for corrosion risk assessment in situations when concrete is wet, dense, or polymer modified, and, thus, the access to oxygen is limited for applying nondestructive evaluation (NDE) methods.(1)
Description
GPM is an electrochemical, NDE technology used for rapid assessment of corrosion and estimation of the corrosion rate of steel rebars. GPM is based on the polarization of a rebar, which is determined by using a small current pulse generated by a counter electrode on the concrete surface and measuring the potential change between the reference electrode and the reinforcement (i.e., the rebar).(2) GPM can determine the HCP, corrosion rate, and resistance between the reinforcement and the device (figure 1).
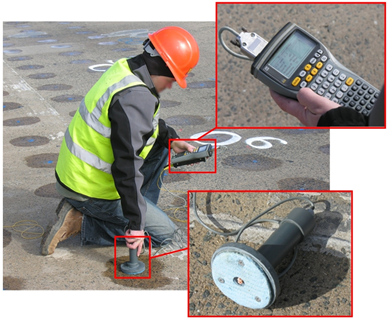
Figure 1. Photo. GPM with closeup view of control unit.(3)
Physical Principle
GPM is based on the measurement of polarization resistance. The anodic side—the location of a corroding rebar—has a surplus of electrons as a result of a release of metal ions into an electrolytically conducting liquid. Those excess electrons flow to the cathodic side, which is concrete with oxidizing agents in the liquid, inducing a corrosion current (Icorr) between the two sides. Knowing the current and voltage allows an inspector to determine the polarization resistance, which is related to the rate of corrosion.
GPM testing is conducted by first applying a number of anodic direct current (DC) pulses
(5–400 μA) to the counter electrode within a confined area. The first pulse is the strongest and lasts between 3 and 60 s; its intensity primarily depends on the properties of the object under investigation. Several smaller consecutive pulses of gradually increasing intensity may follow the first pulse. The objective of applying DC pulses is to obtain a sufficiently high polarization, at which point potential variations are recorded by means of a reference electrode (figure 2). Figure 3 shows the measured potential change (red curve) for a corroded rebar after polarization. Noncorroding or passive reinforcement possesses no current and, thus, will have a high polarization resistance to the current flow.(4) Active corrosion has a smaller polarization resistance. This small polarization can also be explained as an actively corroding system quickly compensating for a removal of electrons owing to the polarization achieved by the applied current pulse. The active Icorr is given by the Stern–Geary equation in figure 4. The corrosion rate can be calculated by the equation in figure 5.
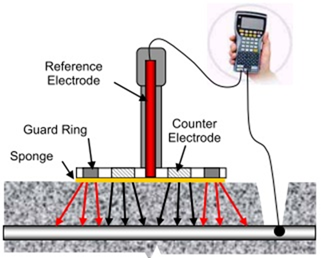
Figure 2. Illustration. GPM of corrosion activity.(4)
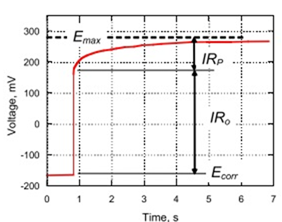
Ecorr= potential of actively corroded rebar; Emax= maximum potential of actively corroded rebar; I = applied pulsed current; R0 = electrical resistance of the concrete cover; RP = polarization resistance of the rebar.
Figure 3. Graph. Potential change after polarization.(5)

Where:
A = confined area (cm2 or inch2).
RP = polarization resistance of the rebar.

Data Acquisition
GPM measurements can be taken at selected locations of a structural element or at gridded points, allowing for fully mapped results. In the latter case, a 2- by 2-ft (0.6- by 0.6-m) grid should be marked on the element using chalk or washable paint. Since the GPM requires the device to be connected to the reinforcing steel, a connection should be made prior to testing by drilling a hole in the concrete element and establishing a connection with a rebar using an alligator clip, screw, or other means (figure 6). Therefore, a rebar locator and drilling equipment should accompany the GPM system. The electrical continuity of the rebar mesh should be checked to identify the need for additional connections to rebars. This check is achieved by measuring resistance between two connections to rebars, typically on diagonally opposite sides of the element. The holes need to be patched with approved material after completing a GPM survey. The surface of the element should be wetted before the survey, or the test locations lightly sprayed shortly before the measurement.
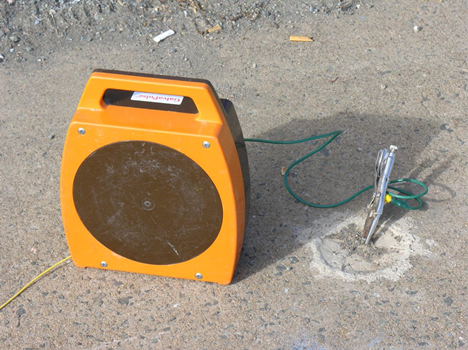
Figure 6. Photo. Connection to an exposed rebar.(3)
The actual measurement is taken by placing a reference electrode on the test location (figure 7). The sponge at the bottom of the electrode should be wet to ensure electrical coupling with the concrete element. One strong and several shorter pulses are applied to achieve sufficiently high polarization of rebars. Potential variations are measured by the reference electrode, and data are recorded on a handheld computer through data acquisition software. Potential changes equal to or higher than 100 mV are indications of passive reinforcement, while lower potential changes are indications of corrosion activity. According to figure 5, the corrosion rate can be calculated because it is proportional to the amount of current required to change the potential.
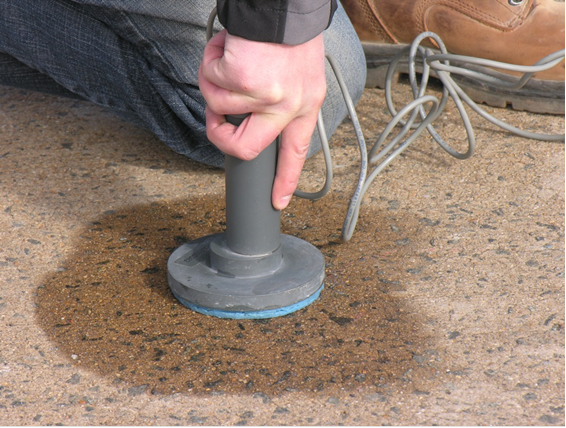
Figure 7. Photo. Reference electrode with wet sponge.(3)
Data Processing
GPM data processing basically involves plotting raw data. No other data processing is required. The equipment usually comes with contour-mapping software by which the raw data are gridded and mapped. The slope of the potential–time curve measured during the current pulse can be used to provide information on the state of rebar corrosion. Potential data can be used to obtain the concrete ER for a given depth of concrete cover.
Data Interpretation
The ER and corrosion rate maps from a GPM survey of a bridge deck are shown in figure 8 and figure 9, respectively. As illustrated in figure 8, the measured ER varies between 5 and 63 kΩ. The lower the ER, the higher the probability of a corrosive environment as a result of the intrusion of moisture, chlorides, and salts into the concrete. On the other hand, highly resistive concrete is an indication of a noncorrosive environment.
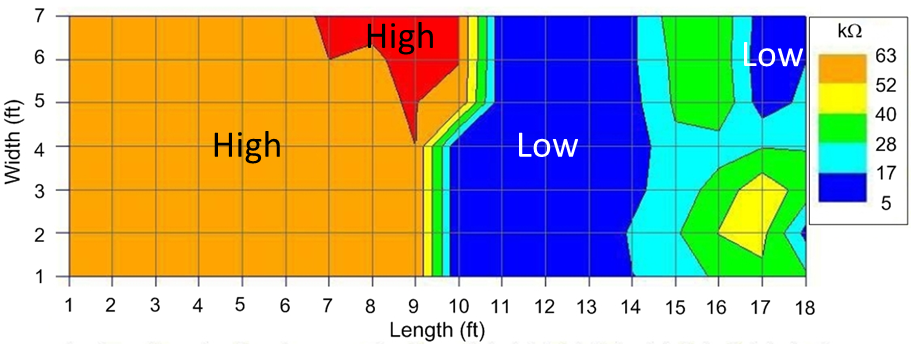
1 ft = 0.3 m.
Figure 8. Contour map. Concrete resistance map as measured by a GPM system.(6)
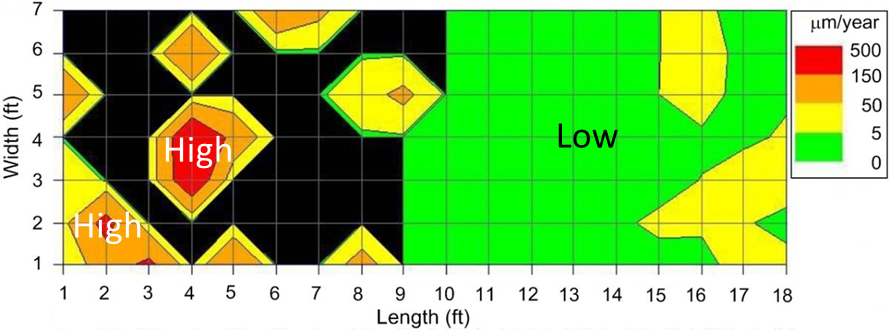
1 ft = 0.3 m; 1 μm = 0.39 mils.
Figure 9. Contour map. Map of corrosion rates.(6)
The calculated corrosion rate can be further correlated to the cross section loss. In figure 9, the cross section loss is calculated and presented in the map with the assumption that a corrosion rate of 1 μA/cm2 (6.45 μA/inch2) corresponds to a cross section loss of about 11.6 μm/yr (0.457 mils/yr).
Advantages
Advantages of GPM include the following:
- Medium level of expertise for equipment setup and data collection.
- Economical test.
- Repeatable test.
- Sufficient accuracy for practical applications.
- HCP and ER determinable.
- Relatively small equipment.
Limitations
Limitations of GPM include the following:
- Direct access to reinforcement necessary.
- Unstable measurements when concrete cover ER is high.
- Prewetting of the measurement area necessary.
- Time consuming and labor intensive for large elements.
- Inaccurate results in aged structures (the change in microstructure of concrete and the loss of moisture from the concrete make the potential of rebar and the ER of concrete more unpredictable).
References
- Sørensen, H.E. and Froelund, T. (2002). “Monitoring of Reinforcement Corrosion in Marine Concrete Structures by the Galvanostatic Pulse Method.” Presented at the International Conference on Concrete in Marine Environments, Hanoi, Vietnam.
- Elsener, B., Hug, A., Bürchler, D., and Böhni, H. (1996). “Evaluation of localized corrosion rate on steel in concrete by galvanostatic pulse technique.” Presented at the Fourth International Symposium Corrosion of Reinforcement in Concrete Construction, London, England.
- Gucunski, N., Imani, A., Romero, F., Nazarian, S., Yuan, D., Wiggenhauser, H., Shokouhi, P., Taffee, A., and Kutrubes, D. (2013). Nondestructive Testing to Identify Concrete Bridge Deck Deterioration, Report No. S2-R06A-RR-1, Transportation Research Board, Washington, DC.
- Baessler, R., Burkert, A., Froelund, T., and Klinghoffer, O. (2003). Usage of GPM Portable Equipment for Determination of Corrosion Stage of Concrete Structures, NACE Corrosion Conference Proceedings, San Diego, CA.
- Germann Instruments. “GalvaPulse.” (website) Evanston, IL. Available online: http://germann.org/products-by-application/half-cell-potential/galvapulse/, last accessed March 11, 2019.
- Strategic Highway Research Program. (n.d.). “SHRP2 NDToolbox.” (website) Washington, DC. Available online: http://www.trb.org/StrategicHighwayResearchProgram2SHRP2/Blank2.aspx, last accessed February 1, 2015.